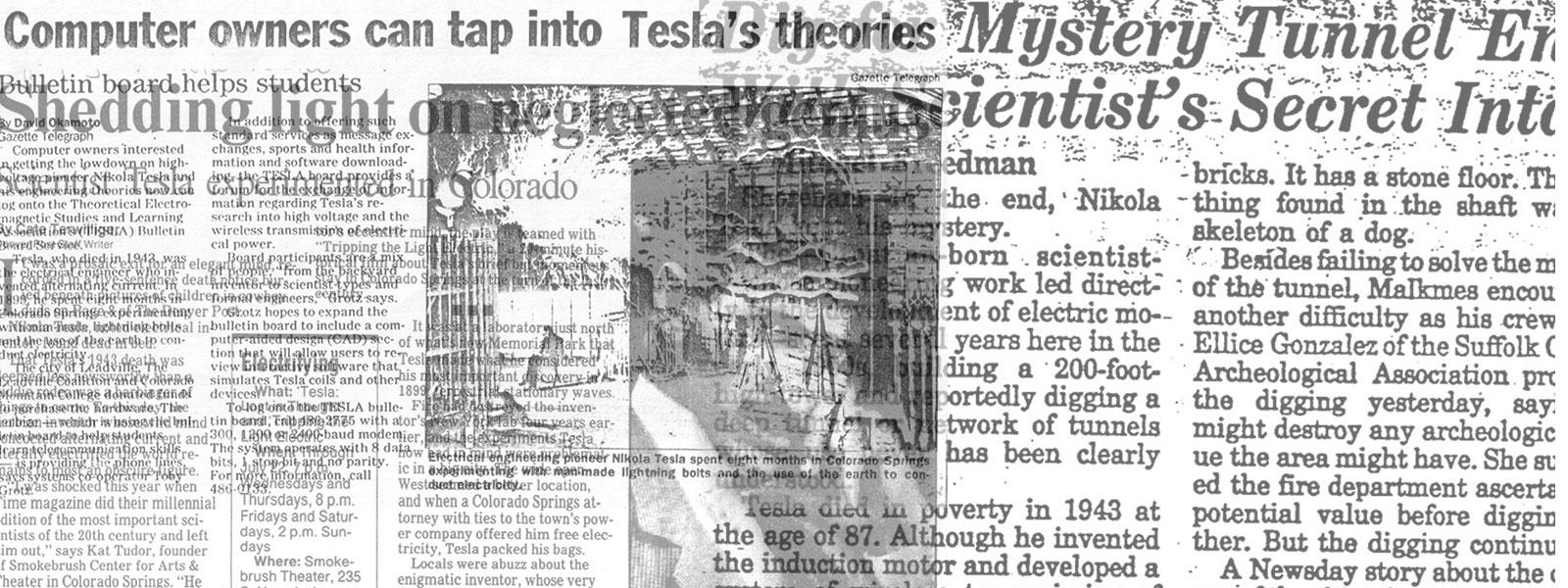
Nikola Tesla Articles
High-Frequency Currents
High-Frequency Currents1
The methods of producing high-frequency currents may be divided broadly into three classes, namely: (1) alternator methods; (2) methods based on the oscillatory discharge of a Leyden jar; (3) arc methods.
In a little special machine which I have here there are 15 magnets - that is to say, 30 poles alternately north and south - and it runs at 8,000 revolutions per minute or 133.3 revolutions per second, so that frequency in this case is 2,000. The peripheral speed of this machine is just over three miles per minute. From this example it will be pretty obvious that if I attempt to obtain high frequencies - that is to say, frequencies well above 10,000 - I shall have to run either at enormous speeds or have a very large number of poles. One of the very earliest high-frequency machines ever constructed in this country, and following shortly after Tesla’s high-frequency machine, was made by Messrs. Pyke and Harris for Sir David Salomons.
The machine ran at 1,500 revolutions per minute, but as both the armature and the field magnets are free to move, their relative rate was 3,000 revolutions per minute, or 50 revolutions per second. The number of pairs of poles is 174, so that frequency is 8,700.
At about the same date that Sir David Salomons had his machine constructed, Mr. Parsons constructed for Professor Ewing at Cambridge University a high-frequency alternator in which the high frequency - namely, 14,000 - was obtained by using a high speed of 12,000 revolutions per minute.
If, instead of moving the wires across the faces of the magnets, or moving the soft iron cores on which they are wound, the wires be wound on the poles of the magnets and a piece of soft iron moved past the magnet poles, an alternating current will be produced as before, with this difference - that for a given number of poles and a given speed this type of alternator will produce twice the frequency of the previous type. Alternators depending on this principle are usually called inductor alternators. Many high-frequency alternators have been constructed on this principle by using 204 polar projections or teeth and a speed of 35,400 revolutions per minute. I have obtained a frequency of no less than 120,000 in a machine I constructed in the laboratories of the Central Technical College, but the output of the machine was microscopic.
Turning to modern high-frequency machines, we have the alternator designed by Mr. Alexanderson, with which frequencies as high as 200,000 have been obtained.
A high-frequency alternator on quite a different principle is due to Dr. Goldschmidt. I have mentioned in Sir David Salomon’s early machine that the two disks forming the field and armature were rotated in opposite directions. It is possible, however, to produce a rotating field electrically without the disk moving at all. When this is accomplished the frequency of the machine depends as before upon the relative speed of the rotating field and the armature.
Consider for simplicity, a machine having only two poles, so that it gives one complete cycle per revolution, and suppose I supply the fixed part or stator with an alternating current of 100 frequency and so connect it that it will produce a rotating field (we will assume the alternating current to be three phase for the sake of simplicity), if now I rotate the rotating part in the opposite direction to that of the rotating field I shall have induced in the rotor an alternating current whose frequency will depend on the relative speeds. For instance, if the speed of the rotor is such that with a stationary field it would give 100 frequency, then as my field is actually rotating in the opposite direction at 100 frequency the actual frequency produced will be 200 per second. Using this 200-per-second frequency by means of another machine running at the same speed one could increase it to 300, and so on indefinitely. This would, however, lead to a very complicated machine, and Dr. Goldschmidt has arranged matters so that instead of requiring a number of machines the successive additions to the frequency can all go on in the same machine.
The simplest way to explain this is to suppose that the alternating current at 200 frequency obtained from the rotor is passed back into the stator of the same machine so as to produce a rotating field of 200 frequency without in any way interfering with the original rotating field of 100 frequency. This rotating field of 200 frequency will induce an alternating current of 300 frequency in the rotor, which could in turn be transferred back to the stator and produce a rotating field having 300 frequency, and so on.
This is what Dr. Goldschmidt calls the electrical method of transferring the currents from the rotor to the stator. He has also devised a magnetic method which is simpler. Turning again to our two-pole machine whose stator is supplied with current at 100 frequency, there will be induced in the rotor a current of 200 frequency. Now, if the rotor be short-circuited there will be produced in it a rotating field having 200 frequency, which will induce in the stator a current having 300 frequency, which in turn can be short-circuited and will induce in the rotor a current having 400 frequency, and so on. I have spoken of the machine as if the rotating field was produced by a three-phase current. A single-phase field may be looked upon, theoretically, as two rotating fields in opposite directions, one of which will have no effect as far as we are concerned, and therefore a single-phase machine will do just as well. Further, it is not really necessary to supply the stator of the machine with an alternating current. If it is excited with a direct current it will induce an alternating current in the rotor which will react on the stator, and backwards and forwards in the same way as I have already described.
It would seem at first sight as if by this means an indefinitely high frequency could be very easily obtained. There are, however, certain practical difficulties.
By this method Dr. Goldschmidt has obtained 12 kilowatts at a frequency of 30,000, and at high frequencies a lesser output.
The present position of the generation of high-frequency currents by means of alternators may be stated by saying that it is now practically possible to generate by means of a machine alternating current up to and over 200,000 frequency. At frequencies of 100,000 several kilowatts are obtainable, and at the lower frequencies machines can be built for greatly increased outputs. Where large amounts of power at high frequencies are required, such as in wireless telegraphy, the question is now becoming a commercial one as to the efficiency of the different types of alternator, their cost and their reliability. Is it possible to run these machines continuously day in and day out and to supply power at high frequencies? If so, how much does it cost per kilowatt-hour to produce this high-frequency power, taking into account not only the coal consumption, but also the wear and tear on the machine and all the other running and capital charges?
Leyden-Jar Discharges
Turning next to the second method of producing high-frequency currents - namely, by the discharge of a Leyden jar - it was quite early inferred by Helmholtz and Henry that the discharge of a Leyden jar did not always flow in the same direction, and we owe to Lord Kelvin the mathematical investigation of this subject, and to Feddersen its experimental demonstration. If I charge a Leyden jar, or any other condenser, and allow it to discharge through a very high resistance, a current will flow, which gradually dies away as the jar discharges. If, however, I discharge the condenser through a sufficiently low resistance, it will be found that the current continues to flow in the same direction after the jar is completely discharged, and then charges it up in the opposite direction, the current gradually getting smaller.
When the jar is charged to a certain extent in the opposite direction to its original direction it starts to discharge again, this time in the opposite direction. The current again continues to flow after the jar is discharged, and charges it up in the original direction. This cycle of operation is repeated, the energy of the charge in the jar gradually getting less and less, until it dies away. The frequency of these oscillations is determined by the capacity of the condenser and the self-induction.
It is quite easy to obtain by this method almost any frequency - for instance, anything between 1 and 100,000,000 are comparatively easily obtainable, and I have even demonstrated frequencies as low as one-half.
The fundamental difference between these high-frequency currents and those generated by alternators is that they die away - that is to say, that the amplitude gradually decreases and comes to zero. The condenser then has to be re-charged, and the process repeats itself. Now, a single discharge of this sort is not of much practical use. It is necessary to charge the condenser and repeat the discharges in rapid succession if any useful work is to be done. Many methods have been proposed for this purpose. Some of the early successful experiments were those of Tesla.
The condenser is allowed to discharge through a circuit which consists of the necessary self-induction and a spark gap in series. The spark gap will stand a certain electrical pressure, and then let the electricity pass. When once the electrical pressure or voltage has been raised to a sufficiently high value to break down the spark gap, the spark gap changes from being an insulator which prevented the flow of electricity to being a comparatively good conductor, and it remains conducting in the ordinary way until the current finally ceases. If the condenser be charged to a sufficiently high voltage, the spark gap will break down, a spark will take place, and during the time that the gap is conducting, oscillations will take place. In order, therefore, to produce the repeated sets of discharges of the condenser, all we have to do is to repeatedly charge the condenser to a sufficiently high voltage, and this is easily accomplished by charging it from a transformer connected to an ordinary low-frequency alternator.
This is the usual method of producing high-frequency currents. It will be evident, however, that the high-frequency currents obtained by this means differ fundamentally from those obtained from an alternator; for instance, with an oscillation frequency of 1,000,000 per second, such as I am using, and 50 oscillations for each discharge of the jar, the whole discharge will last 0.00005 second. As we are only obtaining 170 discharges per second, there is something like 0.005 second between the end of one discharge and the beginning of the next, which is a comparatively long time, about 100 times as long as the oscillations last.
It is obvious that if the discharges can be caused to take place with greater rapidity by using a higher-frequency alternator to charge the jars, the gaps will be closed up, and this is the method originally used by Tesla, and which is now being used in many of the wireless transmitters, though in this case there is another reason for using a greater number of discharges per second. For instance, the Marconi Company, in their musical spark transmitters, use a frequency of some 300 - that is to say, some 600 discharges per second - and the Telefunken Company as many as 1,000 discharges per second.
I now come to another class of method which still depends upon the discharge of a condenser. If I have a body like a pendulum or a spring capable of oscillations, I can make it oscillate by pulling it away from its position of rest and then letting it go. I can also make it oscillate by giving it a blow or shock, and I can make it oscillate by applying to it a number of impulses at properly timed intervals. All three of these methods are employed in the production of high-frequency currents. The first is the simple discharge of the Leyden jar without any auxiliary apparatus; the third is the method usually employed in wireless telegraphy for obtaining and maintaining a long series of oscillations in the aerial by tuning it and loosely coupling it to the Leyden-jar circuit in which the discharge takes place. The second method also involves a discharge of a condenser. In this case the spark gap is so arranged that it suppresses the oscillations of the condenser - that is to say, it breaks down, allows a large rush of current, and then becomes insulating and stops anything further from happening.
It might be thought at first that in this method of producing oscillations there was no relationship between the nature of the swing in the first, or discharge, circuit and the frequency of the oscillations in the second circuit. Without going into the theory of coupling, which would take me too far, I would point out that for the best result there is a relationship, and this can be easily seen if one considers the question of starting oscillations in, say, a short and a long pendulum. The long pendulum will be set swinging easier with a blow which comes on and off comparatively slowly, whereas the short pendulum will require a short, quick tap to start it swinging.
This method of shock excitation, due to Max Wien, is employed by the Telefunken Company in its wireless transmitter, and also in its therapeutic apparatus; a special type of spark gap being used consisting of large flat metal plates, a very short distance apart, the object being to suddenly cool the spark to cause it to go out, and the gap to become insulating again at the earliest possible moment.
A spark gap of this type connected to a condenser and self-induction can be supplied in the ordinary way, when the frequency of the spark will naturally depend upon the frequency of the alternator.
If an attempt be made to employ the successive discharges of a condenser when charged from continuous current, a difficulty is encountered if large powers are dealt with. This difficulty seems to reside in the properties of the spark gap, which tends to become completely conducting, or, what is technically called “arcs,” when large powers, say 10 kilowatts or over, are employed.
Mr. Galletti set himself to get over this difficulty by employing a number of such circuits, each with its own spark gap, and connecting these together so that they would all help to produce the oscillations.
By combining a number of circuits and using the high-voltage continuous current supplied by the Moutier-Lyons transmission - some 40,000 volts - Mr. Galletti was able to deal with many kilowatts, the spark frequency being very high, about 10,000 per second. Corresponding to each of these sparks there is a series of oscillations, so that in this case the oscillations must be completely joined up, and the result almost the same as if an alternator were employed.
Mr. Galletti has been able to employ still larger numbers of sparks per second, up to 100,000. In this case one arrives at the interesting result that the oscillations can be maintained by impulses from the condenser circuit every other oscillation, or even each individual oscillation. This should maintain a true alternating current, such as is produced by an alternator, with no waste intervals.
Arc Methods
If an ordinary direct-current arc is produced between solid carbon or metal electrodes, it is unstable - that is to say, it cannot be burnt unless a resistance is placed in series with it. The current can be kept at any desired value by putting sufficient resistance in series with the arc, so that when the current is increased the potential difference between the terminals of the arc and the resistance taken together increases, and the circuit as a whole becomes stable. If such an arc be shunted with a capacity and self-induction in series oscillations will be set up in this shunt circuit, the frequency of the oscillations being mainly determined by the periodic time of the shunt condenser circuit.
When the oscillations are taking place, the oscillatory current flows through the arc, and consequently tends to increase and decrease the arc current. Any increase in the current tends to make the vapor column of the arc larger, and any decrease makes it smaller, so that the vapor column of the arc pulsates in size. This pulsation in the size of the vapor column moves the air in the neighborhood of the arc, and, if the frequency is suitable, produces sound; hence this experiment is known as the musical arc. By varying either the capacity or the self-induction, the frequency of the oscillations can be varied, and hence the pitch of the notes given out by the arc, and by this means a tune can even be played on it.
The frequency of the currents produced when using an ordinary arc between solid carbon electrodes is comparatively low, at the most a few thousands per second. The reason for this is that the characteristic of the arc - that is to say, the relationship between the potential difference and the current - depends to some extent on how quickly the current is varied. If the current is varied very quickly, then an increase of current is accompanied by an increase in the potential difference; the arc is no longer an unstable conductor, and oscillations cannot be produced.
There are various ways of rendering the arc unstable, even when the current varies rapidly. One of these consists in using very small currents. For instance, metal arcs using currents under one-half ampere are extremely unstable, and remain so even under high frequencies.
Another method is to energetically cool the electrodes; for instance, to make one of the electrodes consist of a vessel containing water, or squirt a jet of water through the arc. The third method, due to Mr. Poulsen, consists in placing the arc in hydrogen, or some hydrogen compound, such as coal gas or alcohol vapor. By this means high-frequency oscillations can easily be produced up to about a million per second, and at the lower frequencies of, say, 100,000, considerable powers can be dealt with.
To render the arc still more unstable, Mr. Poulsen places it in a strong transverse magnetic field. With an apparatus of this sort I am informed that 30 kilowatts or more can be dealt with by a single arc, and if several arcs are placed in series, large powers can be transformed.
If the apparatus is properly adjusted the high-frequency currents produced are very nearly true alternating currents, such as are obtained from an alternator.
The only difficulty I find in working the apparatus is to maintain the frequency absolutely constant, as it appears that the frequency depends to a small extent on the current through the arc and on the arc length. In the actual apparatus employed for wireless telegraphy the electrodes are kept in rotation so as to ensure a greater constancy in the arc length.
In using a generator of this sort for wireless telegraphy, messages have been transmitted and recorded from a distance of about 1,000 miles at the speed of over 100 words per minute.
A large number of modifications of the arc method have been produced, practically all of which consist in changing the nature of the electrodes or the surroundings of the arc, so as to try and make it still more unstable at high frequencies and large currents. Most arcs seem to lose their stability after the current has passed a certain limit. For this reason there is generally a best working current for any arc. If it is required to deal with more power than one arc can transform, it is better to put the arcs in series.
A very interesting generator of high-frequency currents is that invented by S. G. Brown. It is difficult to say whether it is an arc or a spark or what. The unstable conductor which takes the place of the arc consists of an aluminum wheel, on the edge of which a copper block rests. The wheel is kept rotating, and the contact is shunted by a condenser and self-induction. This arrangement produces high-frequency currents quite easily, but I have never been able to make it deal with any large powers.
In conclusion, it is of interest to review in general terms the present position of the three main methods of producing high-frequency currents. The most important application at the moment for high-frequency currents is wireless telegraphy. For this purpose frequencies in the range between 30,000 and 1,000,000 are required. A few kilowatts of high-frequency energy is generally sufficient for practical purposes at the higher frequencies. At the lower frequencies, which are mainly used on long-distance working, large amounts of power are required. In fact, one of the great problems in long-distance wireless telegraphy is to produce several hundred kilowatts of high-frequency energy with certainty and regularity, and at a reasonable price.
The alternator method, as far as the information at my disposal goes, has never yet been applied to very large powers. There is no doubt that it is from many points of view an ideal method.
The spark method has been successfully employed over a wide range of frequency and up to very large powers, and there is no doubt that it is capable of satisfactory operation. The spark method used with very-high-frequency sparks and continuous current, as proposed by Mr. Galletti, is still in its experimental stage. There seems no reason why the principle should not be extended so as to deal with larger powers. The practical difficulties may be great, but I think they should be able to be overcome.
The arc method is in a much more advanced condition. A large amount of work has been done, and practically all the details have been worked out. There is no doubt that fairly large powers can be successfully generated and utilized.
The position is therefore a very interesting one. No one of the methods seems at the moment to have so many advantages as to make it the one method above all others for producing high-frequency currents. The final decision as to which is the best will probably in the end be determined by the regularity with which the apparatus works, and by that governing factor in all commercial enterprises - the cost of production.
1 Portion of an address to the Royal Institution of Great Britain.